Mechanical ventilation plays a critical role in addressing the pathophysiological mechanisms underlying acute respiratory failure (ARF). By correcting gas exchange abnormalities, reducing respiratory muscle workload, and preventing complications, it provides essential support for patients in critical conditions (1,5,7,8). Below, specific mechanisms of mechanical ventilation are linked to the pathophysiological issues they target to enhance clarity and coherence.
Correction of Hypoxemia
One of the primary goals of mechanical ventilation in ARF is to address hypoxemia caused by mechanisms such as ventilation-perfusion (V/Q) mismatch, shunting, or diffusion impairment.
- Mechanism: Mechanical ventilation increases the fraction of inspired oxygen (FiO₂), ensuring higher oxygen availability in the alveoli, which is crucial for patients with reduced oxygenation due to pneumonia, ARDS, or pulmonary embolism.
- PEEP (Positive End-Expiratory Pressure): PEEP prevents alveolar collapse during exhalation by maintaining positive pressure in the lungs, thereby improving functional residual capacity (FRC). This is particularly effective in conditions involving alveolar instability, such as ARDS or atelectasis.
- Link to Pathophysiology: In cases of shunting, where blood bypasses ventilated alveoli, PEEP recruits collapsed alveoli and redistributes ventilation, reducing hypoxemia.
Removal of Hypercapnia
Hypercapnia in ARF arises from hypoventilation or increased dead space, as seen in conditions like COPD exacerbations or severe obesity hypoventilation syndrome.
- Mechanism: Mechanical ventilation increases minute ventilation by optimizing tidal volume (VT) and respiratory rate. By enhancing the removal of carbon dioxide, it normalizes arterial pH and relieves respiratory acidosis.
- Pressure-Control Ventilation (PCV): PCV is particularly beneficial in hypercapnic ARF as it delivers preset pressure during inspiration, ensuring adequate ventilation while minimizing airway pressures.
- Link to Pathophysiology: In hypoventilation-related hypercapnia, increasing ventilatory support reduces carbon dioxide retention, reversing acidemia and preventing hemodynamic instability.
Reduction of Work of Breathing
ARF often places a high workload on respiratory muscles, leading to fatigue and eventual failure, especially in conditions like COPD exacerbations or severe asthma.
- Mechanism: Mechanical ventilation reduces the effort required to breathe by delivering positive pressure during inspiration. Modes like pressure support ventilation (PSV) and assist-control ventilation (ACV) enable respiratory muscle rest while maintaining adequate ventilation.
- Link to Pathophysiology: By decreasing the work of breathing, mechanical ventilation prevents respiratory muscle exhaustion, a key contributor to hypercapnic ARF.
Alveolar Recruitment and Stabilization
Alveolar recruitment strategies are critical for restoring effective gas exchange in conditions like ARDS, where alveolar collapse and severe V/Q mismatch are common.
- Mechanism: PEEP and lung-protective ventilation strategies, such as low tidal volumes, recruit and stabilize alveoli, increasing the surface area available for gas exchange.
- Link to Pathophysiology: In ARDS, where alveolar collapse exacerbates shunting, PEEP opens collapsed alveoli, improving oxygenation and reducing the severity of hypoxemia.
Prevention of Secondary Complications
Mechanical ventilation provides controlled respiratory support that reduces the risk of secondary complications such as aspiration, ventilator-associated pneumonia (VAP), or multi-organ failure.
- Mechanism: Secure airway management with invasive mechanical ventilation protects against aspiration, while precise ventilator settings minimize barotrauma and ventilator-induced lung injury (VILI).
- Link to Pathophysiology: By addressing hypoxemia and hypercapnia, mechanical ventilation prevents systemic complications like hypoxia-induced multi-organ failure and maintains hemodynamic stability.
Mechanical ventilation compensates for the physiological disruptions seen in ARF by targeting specific mechanisms like V/Q mismatch, diffusion impairment, and increased work of breathing. Each intervention, whether optimizing oxygenation, reducing carbon dioxide levels, or stabilizing alveolar function, is tailored to the patient’s underlying pathology.
This ensures effective management of ARF while minimizing complications, emphasizing the indispensable role of mechanical ventilation in critical care settings (1-8,10).
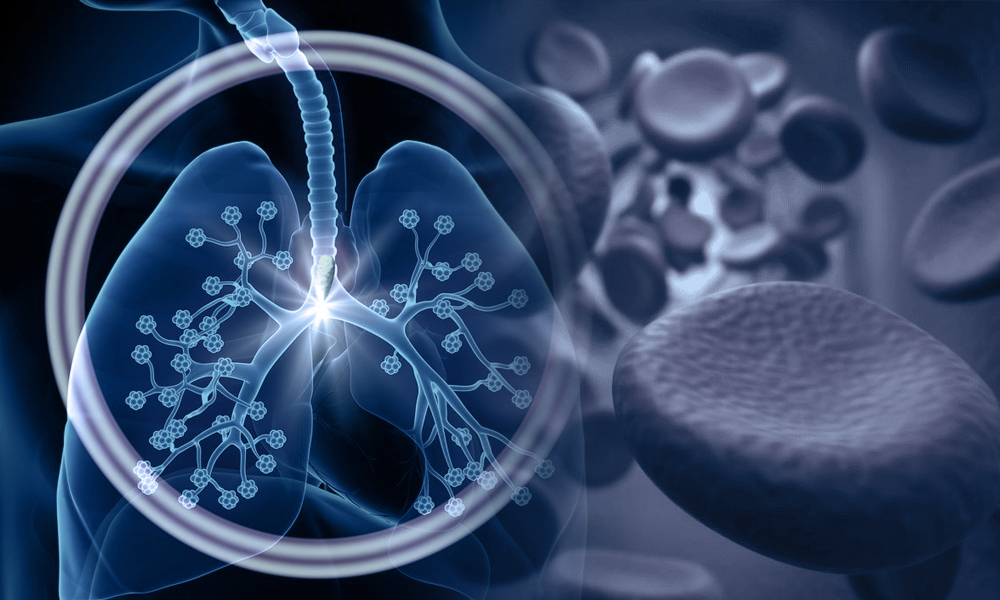
Types of Mechanical Ventilation
Mechanical ventilation is classified into invasive and non-invasive methods, each with specific applications based on the patient’s condition and therapeutic needs (9-11). Both types play a vital role in the management of acute respiratory failure (ARF), with the choice determined by the severity of respiratory dysfunction and the clinical response to treatment (10).
Non-Invasive Ventilation (NIV)
Non-invasive ventilation delivers respiratory support through a mask or nasal interface, avoiding the need for intubation. It is particularly effective in patients with mild to moderate ARF who are conscious and able to protect their airway.
- Mechanism: NIV enhances oxygenation and ventilation using positive airway pressure, which prevents alveolar collapse and reduces the work of breathing.
- Common Modes:
- Continuous Positive Airway Pressure (CPAP): Maintains constant positive airway pressure throughout the respiratory cycle, improving oxygenation in conditions such as cardiogenic pulmonary edema.
- Bilevel Positive Airway Pressure (BiPAP): Alternates between inspiratory positive airway pressure (IPAP) and expiratory positive airway pressure (EPAP), facilitating both oxygenation and ventilation, particularly in hypercapnic ARF seen in COPD exacerbations.
- Clinical Application: NIV is widely used in exacerbations of chronic conditions like COPD, mild ARDS, and heart failure, where it can stabilize gas exchange and reduce the need for intubation.
Invasive Mechanical Ventilation (IMV)
In cases where non-invasive ventilation fails to stabilize ARF or is contraindicated, invasive mechanical ventilation provides controlled respiratory support through an endotracheal or tracheostomy tube.
- Mechanism: IMV directly accesses the airway, ensuring precise delivery of oxygen, removal of carbon dioxide, and reduction of respiratory muscle workload. It also facilitates better protection against aspiration in patients with altered mental status or significant secretions.
- Common Modes:
- Assist-Control Ventilation (ACV): Delivers a preset tidal volume or pressure with every breath, whether initiated by the patient or the ventilator. ACV provides full respiratory support, ideal for patients with severe ARF and minimal respiratory effort.
- Synchronized Intermittent Mandatory Ventilation (SIMV): Combines mandatory ventilator-delivered breaths with the patient’s spontaneous breaths. It is frequently used during weaning to encourage respiratory muscle activity.
- Pressure Support Ventilation (PSV): Supports spontaneous breathing by delivering a set inspiratory pressure. It is commonly employed during recovery or as a step-down mode in the weaning process.
- Clinical Application: IMV is indicated in severe ARF with refractory hypoxemia, hypercapnia unresponsive to NIV, or conditions like ARDS, where invasive strategies like lung-protective ventilation and high PEEP are essential.
Non-invasive ventilation often serves as the first-line treatment in ARF. However, in some cases, such as persistent hypoxemia, rising carbon dioxide levels, or respiratory distress that worsens despite NIV, transitioning to invasive mechanical ventilation becomes necessary to ensure adequate respiratory support and prevent further deterioration (9–11). The specific criteria and clinical scenarios guiding this transition will be examined in a subsequent section.
By tailoring the type of mechanical ventilation to the patient’s condition and response, clinicians can optimize outcomes while minimizing complications.
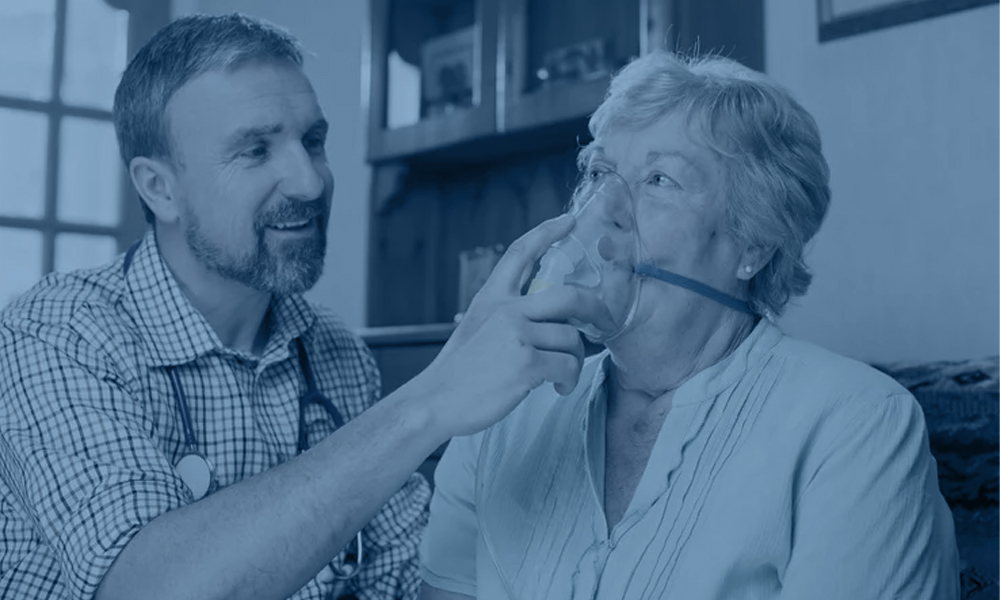
Modes of Mechanical Ventilation for ARF Treatment
The choice of mechanical ventilation mode in acute respiratory failure (ARF) is determined by the underlying pathology, severity of respiratory compromise, and patient-specific factors (11,12). Optimizing the mode of ventilation is essential to ensure adequate gas exchange while minimizing complications (12). Below, the most commonly used modes are outlined, with an emphasis on their applications and clinical significance.
Assist-Control Ventilation (ACV)
ACV provides full respiratory support by delivering a preset tidal volume (VT) or pressure with each breath, regardless of whether the breath is initiated by the patient or the ventilator.
- Application: This mode is typically used in severe ARF, particularly in conditions such as ARDS or pneumonia, where patients may have minimal or absent spontaneous respiratory effort.
- Advantages: Guarantees consistent ventilation and simplifies management in critically ill patients.
Synchronized Intermittent Mandatory Ventilation (SIMV)
SIMV delivers a set number of mandatory breaths, synchronized with the patient’s spontaneous respiratory efforts, allowing for unassisted breaths in between.
- Application: Often employed as an intermediate step in patients transitioning from full ventilatory support to spontaneous breathing, such as during the weaning process.
- Advantages: Promotes respiratory muscle activity while providing a safety net of mandatory ventilator breaths.
Pressure Support Ventilation (PSV)
PSV augments spontaneous breathing by providing a preset level of inspiratory pressure, reducing the effort required for inspiration.
- Application: Commonly used in patients with preserved respiratory drive, such as those recovering from ARF or during non-invasive ventilation (NIV).
- Advantages: Improves patient comfort, reduces work of breathing, and facilitates weaning from invasive ventilation.
Volume-Controlled and Pressure-Controlled Ventilation (VCV/PCV)
- Volume-Controlled Ventilation (VCV) delivers a preset tidal volume, with airway pressure varying depending on lung compliance and resistance.
Application: Used in patients requiring precise control of minute ventilation, such as those with hypercapnia.
- Pressure-Controlled Ventilation (PCV) delivers a preset pressure during inspiration, with tidal volume varying based on lung compliance.
Application: Beneficial in patients with reduced lung compliance, such as in ARDS, to limit peak airway pressures and reduce the risk of barotrauma.
High-Frequency Oscillatory Ventilation (HFOV)
HFOV delivers very small tidal volumes at high frequencies, maintaining continuous positive airway pressure.
- Application: A rescue strategy for refractory hypoxemia in ARDS when conventional ventilation fails.
- Advantages: Minimizes ventilator-induced lung injury (VILI) by preventing alveolar overdistension.
Clinical Significance
The selection of the appropriate mode of mechanical ventilation in ARF is crucial for optimizing outcomes and minimizing complications. For instance, lung-protective ventilation strategies, such as low tidal volume ventilation in ARDS, reduce the risk of ventilator-induced lung injury (11).
Modes like SIMV and PSV play key roles in facilitating weaning and restoring spontaneous respiratory function (11). By tailoring ventilation modes to the individual needs of patients, clinicians can address the specific pathophysiological challenges of ARF while improving survival and recovery rates (11-15).
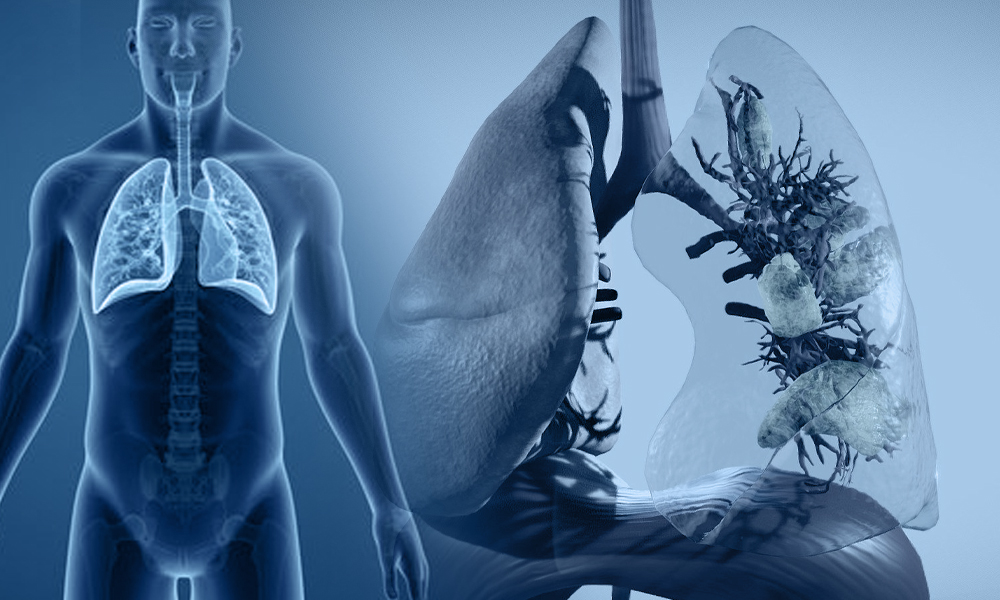
Advanced Modes of Mechanical Ventilation: ASV, SAV, and NAVA
With the evolution of mechanical ventilation, advanced modes like Adaptive Support Ventilation (ASV), SmartCare/Automated Ventilation (SAV), and Neurally Adjusted Ventilatory Assist (NAVA) have emerged as pivotal tools in modern respiratory care. These modes leverage intelligent algorithms and neural control mechanisms to enhance patient outcomes, improve synchronization, and simplify clinical workflows. Below is a comprehensive discussion of each mode and its clinical significance (23-25).
Adaptive Support Ventilation (ASV)
- What is ASV?
ASV is an intelligent mode of mechanical ventilation that automatically adjusts ventilatory support based on the patient’s respiratory mechanics and effort. It uses algorithms to calculate the ideal tidal volume and respiratory rate based on the patient’s predicted body weight, lung compliance, and airway resistance (22-25). - How ASV Works:
- ASV continuously monitors the patient’s spontaneous breathing.
- It dynamically adjusts the inspiratory pressure and ventilatory rate to meet the patient’s metabolic demand while preventing over-distension or atelectasis.
- This mode ensures minimal work of breathing while maintaining target minute ventilation.
- Benefits of ASV:
- Automatically reduces support as the patient’s respiratory effort improves, facilitating a smooth transition from controlled to spontaneous breathing.
- Decreases the risk of hyperventilation, barotrauma, and ventilator-induced lung injury (VILI) (23,24).
- Improves patient-ventilator synchrony, reducing discomfort and sedation requirements (24).
- Evidence and Clinical Applications:
- Studies have shown ASV to be effective in both acute and chronic respiratory failure. For example, research published in Critical Care (Arnal et al., 2008) demonstrated that ASV reduced ventilation duration compared to traditional modes in post-operative ICU patients (26).
- It is widely used in settings requiring dynamic adaptation, such as weaning and managing variable lung mechanics in conditions like ARDS or COPD exacerbations (27).
SmartCare/Automated Ventilation (SAV)
- What is SAV?
SmartCare/Automated Ventilation is a closed-loop ventilation system designed primarily to automate the weaning process. It uses an artificial intelligence algorithm to assess and adjust ventilatory support based on real-time patient parameters (25,28-31). - How SAV Works:
- SAV continuously monitors the patient’s respiratory rate, tidal volume, and end-tidal carbon dioxide (EtCO₂).
- Based on these parameters, it adjusts pressure support levels to ensure adequate ventilation and oxygenation.
- When the algorithm determines that the patient can sustain spontaneous breathing, it suggests readiness for extubation.
- Benefits of SAV:
- Speeds up the weaning process by reducing the need for manual adjustments (22-31).
- Prevents premature or delayed extubation by following evidence-based criteria.
- Reduces the workload for ICU staff and standardizes the weaning process.
- Evidence and Clinical Applications:
- Clinical trials, such as those published in Intensive Care Medicine (Lellouche et al., 2006), have shown that SmartCare reduces the duration of mechanical ventilation and ICU stay while improving extubation success rates (31).
- SAV is especially useful in ICUs with high patient volumes or variability in care protocols (24-31).
Neurally Adjusted Ventilatory Assist (NAVA)
- What is NAVA
Neurally Adjusted Ventilatory Assist (NAVA) is an advanced mode of mechanical ventilation that directly uses the patient’s neural respiratory drive to control ventilatory support. Unlike traditional modes that rely on airway pressure or flow triggers, NAVA is driven by electrical activity in the diaphragm (Edi), offering highly personalized and precise ventilation (25-34).
- How NAVA Works?
Edi Catheter:
A specialized nasogastric catheter detects electrical signals from the diaphragm during the patient’s spontaneous breathing efforts.
Signal Processing:
These signals (Edi) are analyzed in real-time to determine the timing, duration, and intensity of inspiratory effort.
Ventilator Response:
The ventilator delivers pressure support proportional to the patient’s effort, ensuring optimal synchronization and avoiding over-assistance or under-assistance. - Advantages of NAVA
Improved Patient-Ventilator Synchrony:
NAVA directly responds to the patient’s neural respiratory signals, eliminating delays and mismatches that occur with pressure or flow triggering.
Reduces asynchrony-related complications, such as ineffective efforts, double triggering, and patient discomfort.
Reduction in Sedation Needs:
By providing natural, synchronized support, NAVA minimizes the discomfort associated with mechanical ventilation, reducing the need for sedatives.
Enhanced Respiratory Muscle Protection:
Unlike traditional modes that can over-assist, leading to respiratory muscle atrophy, NAVA ensures the diaphragm remains active, maintaining muscle strength and endurance.
Personalized Ventilation:
Adapts dynamically to the patient’s changing respiratory demands, particularly in conditions like ARDS, COPD, or neuromuscular disorders.
- Clinical Applications of NAVA
ARDS and Acute Respiratory Failure (ARF):
NAVA provides lung-protective ventilation by avoiding overdistension and barotrauma while maintaining synchrony (38).
Neonatal and Pediatric Ventilation:
Especially useful in premature infants and pediatric patients, where synchrony challenges are common with traditional modes (36-37,39-43).
Weaning:
Facilitates a gradual reduction in ventilatory support by responding to the patient’s natural efforts (44-45).
NAVA represents a significant advancement in mechanical ventilation, focusing on precision and patient-centric care by using the diaphragm’s electrical activity to guide support. Its ability to enhance patient-ventilator synchrony, reduce sedation needs, and protect respiratory muscles makes it an invaluable mode in both adult and pediatric populations. When combined with other modes like ASV and SAV, NAVA provides a versatile and comprehensive approach to managing acute respiratory failure.
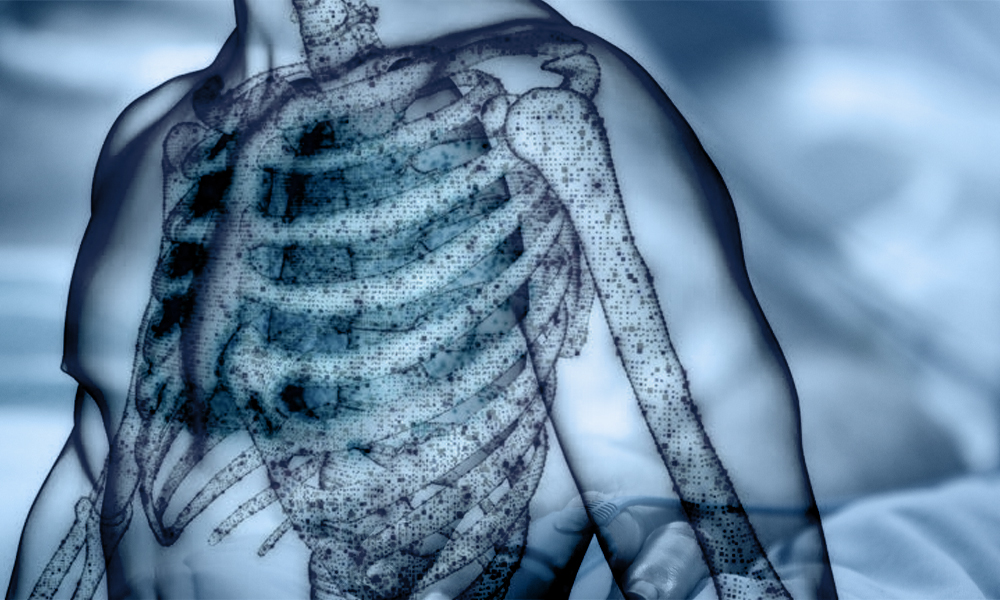
When to Transition from Non-Invasive Mechanical Ventilation (NIMV) to Invasive Mechanical Ventilation (IMV) in ARF
Non-invasive mechanical ventilation (NIMV) is often the first-line treatment for acute respiratory failure (ARF) in specific conditions like COPD exacerbations or mild-to-moderate hypoxemia. However, in some cases, NIMV may fail to provide adequate support, requiring a transition to invasive mechanical ventilation (IMV) to prevent further deterioration. Here are the key indicators and clinical scenarios to consider transitioning:
1. Worsening Gas Exchange Despite NIMV
- Signs:
- Persistent or worsening hypoxemia (PaO₂ < 60 mmHg on FiO₂ ≥ 0.6).
- Rising PaCO₂ (> 50 mmHg) with associated respiratory acidosis (pH < 7.25).
- Reason: Indicates that NIMV is insufficient to meet oxygenation or ventilation demands.
2. Severe Respiratory Distress
- Signs:
- Increased respiratory rate (> 35 breaths/min).
- Use of accessory muscles, paradoxical breathing, or nasal flaring.
- Inability to speak in full sentences due to dyspnea.
- Reason: Suggests that the patient’s work of breathing has exceeded the support provided by NIMV, risking respiratory muscle fatigue and collapse.
3. Altered Mental Status
- Signs:
- Confusion, agitation, or inability to cooperate with NIMV.
- Progression to somnolence or coma (indicating hypercapnia or hypoxemia affecting cerebral function).
- Reason: Neurological impairment reduces the ability to maintain spontaneous breathing or adhere to NIMV.
4. Hemodynamic Instability
- Signs:
- Hypotension (systolic blood pressure < 90 mmHg) unresponsive to fluid resuscitation.
- Shock or signs of reduced cardiac output.
- Reason: Indicates systemic compromise that requires better oxygenation and ventilation control via IMV.
5. Risk of Aspiration or Airway Protection Issues
- Signs:
- Inability to clear secretions or significant mucus plugging.
- Frequent vomiting or risk of aspiration (e.g., altered consciousness).
- Reason: IMV is necessary to secure the airway with an endotracheal tube to prevent aspiration-related complications.
6. Failure to Tolerate NIMV
- Signs:
- Poor mask seal due to facial anatomy or agitation.
- Skin breakdown or discomfort causing discontinuation of therapy.
- Reason: Intolerance to NIMV can reduce adherence, rendering it ineffective.
7. No Clinical Improvement Within 1–2 Hours of NIMV
- Signs:
- Lack of improvement in respiratory rate, gas exchange, or oxygenation after 1–2 hours of optimal NIMV.
- Reason: Delaying the switch to IMV increases the risk of respiratory arrest and worsens outcomes.
The decision to switch from NIMV to IMV in ARF should be guided by clinical judgment, monitoring of gas exchange, respiratory mechanics, and overall patient stability. Early recognition of NIMV failure and timely intubation improve outcomes and prevent complications associated with delayed intervention (12-14, 16-21).
References
- Kapil S, Wilson JG. Mechanical Ventilation in Hypoxemic Respiratory Failure. Emerg Med Clin North Am. 2019;37(3):431-444. doi:10.1016/j.emc.2019.04.005
- Delerme S, Ray P. Acute respiratory failure in the elderly: diagnosis and prognosis. Age Ageing. 2008;37(3):251-257. doi:10.1093/ageing/afn060
- Antro C, Merico F, Urbino R, Gai V. Non-invasive ventilation as a first-line treatment for acute respiratory failure: “real life” experience in the emergency department. Emerg Med J. 2005;22(11):772-777. doi:10.1136/emj.2004.018309
- Piraino T. Noninvasive Respiratory Support in Acute Hypoxemic Respiratory Failure. Respir Care. 2019;64(6):638-646. doi:10.4187/respcare.06735
- Abellan C, Bertin C, Fumeaux T, Carrel L. Insuffisance respiratoire aiguë : prise en charge hospitalière non invasive [Acute respiratory failure : non-invasive hospital management]. Rev Med Suisse. 2020;16(705):1636-1644.
- Munshi L, Mancebo J, Brochard LJ. Noninvasive Respiratory Support for Adults with Acute Respiratory Failure. N Engl J Med. 2022;387(18):1688-1698. doi:10.1056/NEJMra2204556
- Pisani L, Corcione N, Nava S. Management of acute hypercapnic respiratory failure. Curr Opin Crit Care. 2016;22(1):45-52. doi:10.1097/MCC.0000000000000269
- Liu YJ, Zhao J, Tang H. Non-invasive ventilation in acute respiratory failure: a meta-analysis. Clin Med (Lond). 2016;16(6):514-523. doi:10.7861/clinmedicine.16-6-514
- Pham T, Brochard LJ, Slutsky AS. Mechanical Ventilation: State of the Art. Mayo Clin Proc. 2017;92(9):1382-1400. doi:10.1016/j.mayocp.2017.05.004
- Tobin MJ, Laghi F, Jubran A. Ventilatory failure, ventilator support, and ventilator weaning. Compr Physiol. 2012;2(4):2871-2921. doi:10.1002/cphy.c110030
- Tobin, M. J. (2013). Principles and practice of mechanical ventilation, 3e. McGraw-Hill Education LLC.
- Pinsky MR. Toward a better ventilation strategy for patients with acute lung injury. Crit Care. 2000;4(4):205-206. doi:10.1186/cc695
- Rochwerg B, Brochard L, Elliott MW, et al. Official ERS/ATS clinical practice guidelines: noninvasive ventilation for acute respiratory failure. Eur Respir J. 2017;50(2):1602426. Published 2017 Aug 31. doi:10.1183/13993003.02426-2016
- Torres A, Niederman MS, Chastre J, et al. International ERS/ESICM/ESCMID/ALAT guidelines for the management of hospital-acquired pneumonia and ventilator-associated pneumonia: Guidelines for the management of hospital-acquired pneumonia (HAP)/ventilator-associated pneumonia (VAP) of the European Respiratory Society (ERS), European Society of Intensive Care Medicine (ESICM), European Society of Clinical Microbiology and Infectious Diseases (ESCMID) and Asociación Latinoamericana del Tórax (ALAT). Eur Respir J. 2017;50(3):1700582. Published 2017 Sep 10. doi:10.1183/13993003.00582-2017
- Oczkowski S, Ergan B, Bos L, et al. ERS clinical practice guidelines: high-flow nasal cannula in acute respiratory failure. Eur Respir J. 2022;59(4):2101574. Published 2022 Apr 14. doi:10.1183/13993003.01574-2021
- Luo F, Annane D, Orlikowski D, et al. Invasive versus non-invasive ventilation for acute respiratory failure in neuromuscular disease and chest wall disorders. Cochrane Database Syst Rev. 2017;12(12):CD008380. Published 2017 Dec 4. doi:10.1002/14651858.CD008380.pub2
- Blumenthal JA, Duvall MG. Invasive and noninvasive ventilation strategies for acute respiratory failure in children with coronavirus disease 2019. Curr Opin Pediatr. 2021;33(3):311-318. doi:10.1097/MOP.0000000000001021
- Shang P, Zhu M, Baker M, Feng J, Zhou C, Zhang HL. Mechanical ventilation in Guillain-Barré syndrome. Expert Rev Clin Immunol. 2020;16(11):1053-1064. doi:10.1080/1744666X.2021.1840355
- Davidson AC, Banham S, Elliott M, et al. BTS/ICS guideline for the ventilatory management of acute hypercapnic respiratory failure in adults [published correction appears in Thorax. 2017 Jun;72(6):588. doi: 10.1136/thoraxjnl-2015-208209corr1]. Thorax. 2016;71 Suppl 2:ii1-ii35. doi:10.1136/thoraxjnl-2015-208209
- Kress JP, O’Connor MF, Schmidt GA. Clinical examination reliably detects intrinsic positive end-expiratory pressure in critically ill, mechanically ventilated patients. Am J Respir Crit Care Med. 1999;159(1):290-294. doi:10.1164/ajrccm.159.1.9805011
- Rittayamai N, Katsios CM, Beloncle F, Friedrich JO, Mancebo J, Brochard L. Pressure-Controlled vs Volume-Controlled Ventilation in Acute Respiratory Failure: A Physiology-Based Narrative and Systematic Review. Chest. 2015;148(2):340-355. doi:10.1378/chest.14-3169
- Brunner JX, Iotti GA. Adaptive Support Ventilation (ASV). Minerva Anestesiol. 2002;68(5):365-368.
- Dai YL, Hsu RJ, Huang HK, et al. Adaptive support ventilation attenuates postpneumonectomy acute lung injury in a porcine model. Interact Cardiovasc Thorac Surg. 2020;31(5):718-726. doi:10.1093/icvts/ivaa157
- Buiteman-Kruizinga LA, Mkadmi HE, Schultz MJ, Tangkau PL, van der Heiden PLJ. Comparison of Mechanical Power During Adaptive Support Ventilation Versus Nonautomated Pressure-Controlled Ventilation-A Pilot Study. Crit Care Explor. 2021;3(2):e0335. Published 2021 Feb 15. doi:10.1097/CCE.0000000000000335
- Fernández J, Miguelena D, Mulett H, Godoy J, Martinón-Torres F. Adaptive support ventilation: State of the art review. Indian J Crit Care Med. 2013;17(1):16-22. doi:10.4103/0972-5229.112149
- Arnal JM, Wysocki M, Nafati C, et al. Automatic selection of breathing pattern using adaptive support ventilation. Intensive Care Med. 2008;34(1):75-81. doi:10.1007/s00134-007-0847-0
- Zhu F, Gomersall CD, Ng SK, Underwood MJ, Lee A. A randomized controlled trial of adaptive support ventilation mode to wean patients after fast-track cardiac valvular surgery. Anesthesiology. 2015;122(4):832-840. doi:10.1097/ALN.0000000000000589
- Rose L, Schultz MJ, Cardwell CR, Jouvet P, McAuley DF, Blackwood B. Automated versus non-automated weaning for reducing the duration of mechanical ventilation for critically ill adults and children. Cochrane Database Syst Rev. 2014;2014(6):CD009235. Published 2014 Jun 10. doi:10.1002/14651858.CD009235.pub3
- Rose L, Schultz MJ, Cardwell CR, Jouvet P, McAuley DF, Blackwood B. Automated versus non-automated weaning for reducing the duration of mechanical ventilation for critically ill adults and children: a cochrane systematic review and meta-analysis. Crit Care. 2015;19(1):48. Published 2015 Feb 24. doi:10.1186/s13054-015-0755-6
- Wysocki M, Jouvet P, Jaber S. Closed loop mechanical ventilation. J Clin Monit Comput. 2014;28(1):49-56. doi:10.1007/s10877-013-9465-2
- Lellouche F, Mancebo J, Jolliet P, et al. A multicenter randomized trial of computer-driven protocolized weaning from mechanical ventilation. Am J Respir Crit Care Med. 2006;174(8):894-900. doi:10.1164/rccm.200511-1780OC
- Shah SD, Anjum F. Neurally Adjusted Ventilatory Assist (NAVA). In: StatPearls. Treasure Island (FL): StatPearls Publishing; May 22, 2023.
- Navalesi P, Longhini F. Neurally adjusted ventilatory assist. Curr Opin Crit Care. 2015;21(1):58-64. doi:10.1097/MCC.0000000000000167
- Sheng Y, Shao W, Wang Y, Kang X, Hu R. Zhonghua Wei Zhong Bing Ji Jiu Yi Xue. 2023;35(11):1229-1232. doi:10.3760/cma.j.cn121430-20230222-00101
- Vahedi NB, Ramazan-Yousif L, Andersen TS, Jensen HI. Implementation of Neurally Adjusted Ventilatory Assist (NAVA): Patient characteristics and staff experiences. J Healthc Qual Res. 2020;35(4):253-260. doi:10.1016/j.jhqr.2020.03.008
- Mandyam S, Qureshi M, Katamreddy Y, et al. Neurally Adjusted Ventilatory Assist Versus Pressure Support Ventilation: A Comprehensive Review. J Intensive Care Med. 2024;39(12):1194-1203. doi:10.1177/08850666231212807
- Sugunan P, Hosheh O, Garcia Cusco M, Mildner R. Neurally-Adjusted Ventilatory Assist (NAVA) versus Pneumatically Synchronized Ventilation Modes in Children Admitted to PICU. J Clin Med. 2021;10(15):3393. Published 2021 Jul 30. doi:10.3390/jcm10153393
- Umbrello M, Antonucci E, Muttini S. Neurally Adjusted Ventilatory Assist in Acute Respiratory Failure-A Narrative Review. J Clin Med. 2022;11(7):1863. Published 2022 Mar 28. doi:10.3390/jcm11071863
- Beck J, Emeriaud G, Liu Y, Sinderby C. Neurally-adjusted ventilatory assist (NAVA) in children: a systematic review. Minerva Anestesiol. 2016;82(8):874-883.
- Minamitani Y, Miyahara N, Saito K, Kanai M, Namba F, Ota E. Noninvasive neurally-adjusted ventilatory assist in preterm infants: a systematic review and meta-analysis. J Matern Fetal Neonatal Med. 2024;37(1):2415373. doi:10.1080/14767058.2024.2415373
- Fang SJ, Su CH, Liao DL, et al. Neurally adjusted ventilatory assist for rapid weaning in preterm infants. Pediatr Int. 2023;65(1):e15360. doi:10.1111/ped.15360
- Rossor TE, Hunt KA, Shetty S, Greenough A. Neurally adjusted ventilatory assist compared to other forms of triggered ventilation for neonatal respiratory support. Cochrane Database Syst Rev. 2017;10(10):CD012251. Published 2017 Oct 27. doi:10.1002/14651858.CD012251.pub2
- Stein H, Beck J, Dunn M. Non-invasive ventilation with neurally adjusted ventilatory assist in newborns. Semin Fetal Neonatal Med. 2016;21(3):154-161. doi:10.1016/j.siny.2016.01.006
- Liu L, Xu X, Sun Q, et al. Neurally Adjusted Ventilatory Assist versus Pressure Support Ventilation in Difficult Weaning: A Randomized Trial. Anesthesiology. 2020;132(6):1482-1493. doi:10.1097/ALN.0000000000003207
- Yuan X, Lu X, Chao Y, et al. Neurally adjusted ventilatory assist as a weaning mode for adults with invasive mechanical ventilation: a systematic review and meta-analysis. Crit Care. 2021;25(1):222. Published 2021 Jun 29. doi:10.1186/s13054-021-03644-z